Advanced Nucleic Acid Testing Methods Rise to the Challenge
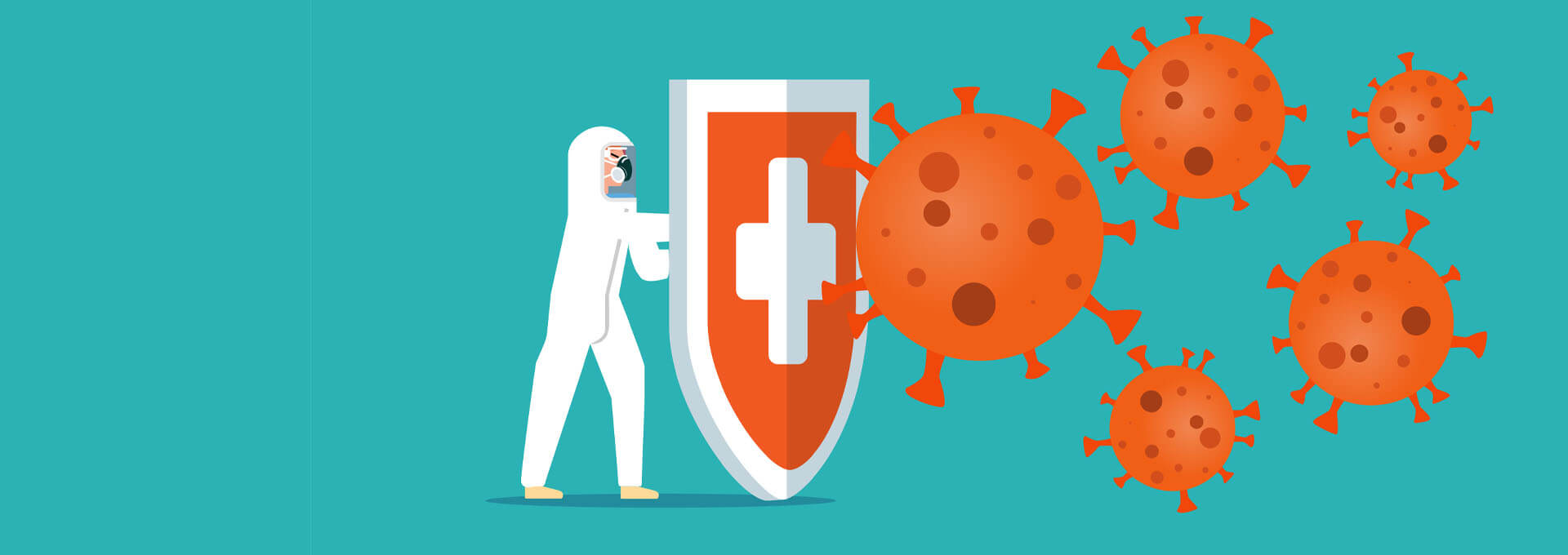
Technological advancements have accelerated the growth of the biopharmaceutical industry and resulted in an increase in the number and diversity of new biologic products in recent years (1). While these products are vital and beneficial to patients, the process of manufacturing biopharmaceuticals carries an inherent risk of microbial and viral contamination.
Consequently, extensive preventative actions have been implemented to mitigate the risk of infecting patients. Adventitious viruses, in particular, can be difficult to detect in these products and have reportedly contaminated various biologic production processes, leading to expensive and time-consuming manufacturing holds (2). Biologic products' safety depends upon a multi-tiered viral safety program that requires starting material control, viral inactivation or removal steps within manufacturing processes, and robust viral detection methods along the entire manufacturing process (3,4). Traditionally, broad-based viral detection has been performed using imaging, culture-based infectivity, and in vivo infectivity techniques (see Table 1). Specific virus families may also be detected using targeted approaches such as assays to detect enzymatic activity unique to the virus type (e.g., retroviral reverse transcriptase). Unfortunately, some adventitious viruses can be difficult to detect using these traditional methods, and regulatory agencies have increasingly recommended alternative virus-specific detection methods, like polymerase chain reaction (PCR), to detect specific viruses that present a risk to biomanufacturing processes (4,5,6,7). Innovations in advanced nucleic acid testing (NAT) methods have enabled the development of assays with broad-based virus detection capabilities while maintaining high sensitivity. These technologies have the capacity to resolve many of the challenges presented by emerging cell and gene therapy (CGT) modalities, which often have unique viral contamination risks that are difficult to address with conventional viral risk mitigation strategies. Here, we discuss those challenges and elaborate on how new viral detection technologies promise to provide solutions, and we also discuss the regulatory expectations for incorporating novel technologies into a comprehensive viral safety program.
In addition, we provide examples where emerging technologies for virus detection can also be applied to help accelerate biologics development in general as the industry changes to increase productivity and utilize alternative manufacturing processes, as well as to address concerns over the use of animal-based testing by incorporating alternative testing approaches wherever possible that are in line with the 3Rs (replacement, reduction, and refinement) philosophy.
Table 1 Summary of virus detection technologiesAssay Type | Type of Assay | Description | Advantages | Disadvantages |
---|---|---|---|---|
In Vivo | Detection of infectious virus | In vivo assays use a variety of different species to enable the detection of a broad spectrum of infectious viruses. Different endpoints can be used to assess viral infection (animal health, antibody production). | Detects infectious virus | Ethical concerns, sensitivity, unable to validate, assay variability, and lack of robustness. |
In Vitro | Detection of infectious virus | Cell culture-based assays using detector cell lines and a variety of endpoints to enable broad spectrum detection of possible adventitious virus (e.g., detection of CPE, immunofluorescence specific for viral proteins, hemagglutination, and hemadsorption). | Detects infectious virus and adaptable for different virus risk factors based on detector cell line selection. | Not all viruses can be cultured in vitro and only a small number of cell lines can be included for routine testing (for practicality). |
Transmission Electron Microscopy | Direct visualization of viral particles | High magnification microscopy technique to generate images based on transmission of electrons through a thin sample section. | Direct detection of virus particles. Morphology observed can help identify viral contaminant. | Does not indicate whether viral particles detected are infectious. Low sensitivity. Not widely accessible due to specialized instrument and expertise required. |
Simple PCR | NAT-detection of viral nucleic acid | PCR assay to amplify short sequence of nucleic acid specific to the virus/virus family contaminant. | Highly specific and high sensitivity to address individual virus contamination risk. | Prior knowledge of targets required, does not indicate whether nucleic acid detected is associated with infectious virus contamination. |
Advanced PCR | NAT-detection of viral nucleic acid | Multiplex PCR utilizing multiple degenerate primer sets, each targeting a different viral family or variant. | Multiplexed assays using degenerate primer design can greatly extend the range of viruses and variants detected, making this approach a viable alternative for existing broad-spectrum virus detection assays.
Rapid compared with traditional methods. | Prior knowledge of target virus families required, does not indicate whether the nucleic acid detected is associated with infectious virus contamination. |
NGS | NAT-detection of viral nucleic acid | Nucleic acid from a quality control (QC) test sample (cell bank, harvest) is sequenced using high throughput sequencing techniques following the construction of a sequencing library. Viral nucleic acid is detected by comparison of sequence data generated with database information to confirm viral origin. | No prior knowledge of virus contaminant required. Sample processing is rapid compared with traditional methods. | Does not directly indicate whether the nucleic acid detected is associated with infectious virus contamination. However, sequencing data can be further assessed to evaluate whether there is evidence of replicating virus (e.g., genome replication
intermediates)
Considerable expertise is required to evaluate data. |
Novel Modality Viral Safety Challenges
CGTs have a variety of challenges that can make establishing a multi-tiered viral safety program difficult. One of the most glaring challenges is the inability to perform virus inactivation or removal steps during the downstream processing of most CGT therapy products (see Figure 1). The downstream processing of recombinant proteins usually includes virus inactivation, filtration, and several chromatography steps that remove potential virus contaminants (see Figure 1a). In contrast, many CGT products cannot use the same techniques without destroying or removing the intended product (see Figure 1b). There has been little regulatory guidance regarding the inclusion of virus removal methods in CGT manufacturing processes. However, the ICH Q5A (R2): Viral Safety Evaluation of Biotechnology Products Derived from Cell Lines of Human or Animal Origin revision incorporates new guidance to include adventitious virus removal steps and virus clearance validation studies to assess effectiveness for adeno-associated virus vectors and other non-enveloped vector-based products (4). For those CGT products that cannot incorporate virus removal procedures during manufacturing, the other two pillars of a multi-tiered viral safety program, raw material control, and product testing, become even more imperative. Unfortunately, many CGT modalities may also find raw material control and product testing challenging.
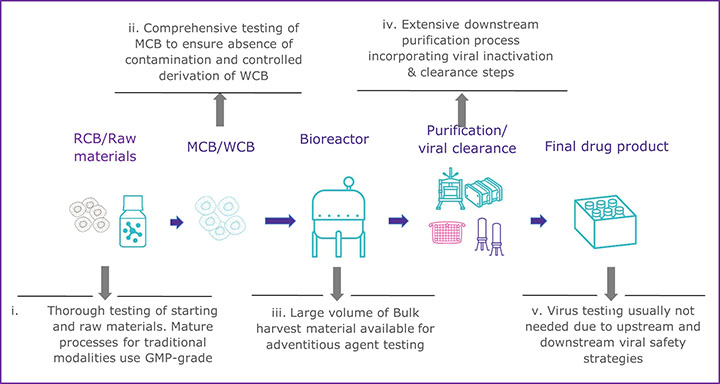
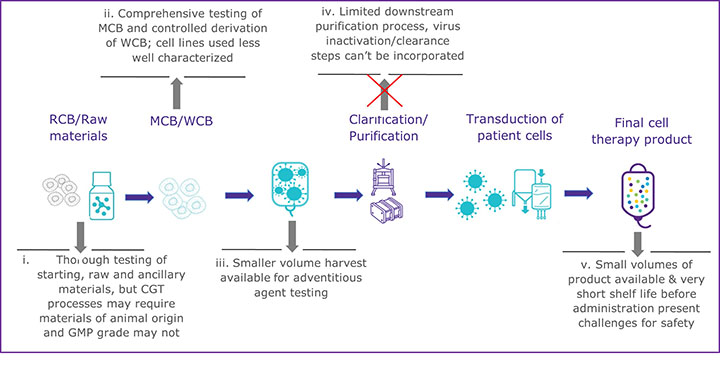
To prevent viral contamination during biopharmaceutical production, appropriately sourced and vetted raw and starting materials should be used, but due to the innovative nature of many CGTs, that can be difficult. Expression systems, starting, and raw materials are often chosen based on active properties rather than quality standards in the early phases of development, according to Figure 1b. If raw materials of appropriate quality cannot be used, there is a greater risk to the final product unless a comprehensive qualification and testing program is implemented. All starting materials should undergo a thorough viral contamination risk assessment that includes evaluating whether the material has come in contact with human- or animal-derived materials (4,8). Depending on the risk identified, virus testing should be conducted before the material is used to generate the clinical product. When there is a known risk of contamination from a specific virus, consider the following:
- Virus-specific detection methods with greater sensitivity should be used to complement any broad-based viral detection methods.
- Broad-based viral detection methods should be qualified to show they meet sensitivity expectations for the particular contaminating viral risk in the relevant matrices.
Meeting the high safety expectations for starting materials will reduce the risk of viral contamination to the final product and, therefore, help to reduce any excessive biosafety testing downstream.
The last pillar of a comprehensive viral safety program requires robust product screening at different stages of the manufacturing process. However, this, too, can become challenging for many CGT products, which often have small batch sizes and require rapid release. Due to the larger volumes and longer test durations required, these challenges often cannot be met using traditional virus detection methods. In addition to the challenges to virus testing presented by CGT products, there are other situations where traditional virus detection methods may be limited. Situations such as the recent COVID-19 pandemic, for example, highlighted the need for accelerated development timelines for both vaccines and therapies, and the use of alternative and rapid NAT methods may enable faster viral safety assessments at multiple quality control testing points. Other changes in the industry, including a shift towards intensified and continuous manufacturing, also increase the need for rapid testing methods to enable faster product release. Therefore, to overcome the challenges faced by many CGT products and accelerated development timelines, newer NAT methods may be appropriate to replace or complement the traditional virus detection methods to build a comprehensive viral risk evaluation and safety program.
Solving Virus Safety Testing Challenges Using Novel Viral Detection Technologies
NAT methods, such as next-generation sequencing (NGS) and advanced, degenerate PCR, can resolve many adventitious virus testing challenges and are increasingly promoted to replace conventional techniques to meet global industry objectives to replace, reduce, and refine the use of animal testing. While these advanced NAT methods can offer broad-based and sensitive virus detection, these techniques also often require less sample volume and can provide a rapid testing solution. Consequently, these NAT methods can be applied to various sample types to complement and sometimes replace traditional viral detection methods.
Likewise, advanced PCR methods can be designed to detect various virus variants within different viral families in a single reaction by using multiple degenerate primer sets. These multiplexed, degenerate PCR methods, therefore, can provide a level of broad detection capabilities while still maintaining the high sensitivity associated with PCR due to the many rounds of target amplification. Unlike NGS, multiplexed degenerate PCR methods will retain some detection bias owing to design parameters. However, the enhanced sensitivity of PCR methods often compensates for decreased detection breadth. Therefore, those sample types with a narrower viral risk profile may find advanced PCR methods that ensure lower limits of viral detection are met more appropriate than NGS. While an NGS method can be qualified to show it meets viral detection sensitivity in the relevant matrix via a sample spiking study, the spiking study itself can consume precious material. However, both NAT methods can be performed with significantly less volume than traditional culture-based methods. The decision to replace or complement a traditional virus detection method with degenerate PCR or NGS should be considered on a case-by-case basis dependent on a comprehensive viral risk assessment.
Regulatory Acceptance of Novel Viral Detection Methods
Although some broad-based NAT methods have been available for almost two decades, their adoption by the biopharmaceutical industry for quality control purposes has been slow. There is a perception that replacing compendial or well-established methods would invite regulatory scrutiny and potentially delay product approvals. Developing, publishing, and updating regulatory guidance can be a multi-year process, and guidance can only be based on technologies and knowledge current at the time of writing. However, most guidance often includes permissive language to use alternate technologies. For example, ICH Q5A states, "Since the most appropriate techniques may change with scientific progress, proposals for alternative techniques, when accompanied by adequate supporting data, may be acceptable." Therefore, regulators expect that an alternative method be demonstrated as being fit for purpose and equivalent or better than conventional methods. This equates to ensuring that the following method has been followed:
- Validated according to ICH Q2 (R1): Validation of Analytical Procedures principles or another appropriate local guidance (9).
- Shown to be suitable for the specific sample matrix to be tested.
- Demonstrated to have equal or better performance through a comparability exercise.
However, the ICH Q5A revision clarifies that a head-to-head comparison for the replacement of an in vivo method is not required, and regional regulatory agencies are expected to implement this approach.
To incorporate an alternative viral detection method, manufacturers should perform a risk assessment, develop a strategy, and ensure that regulatory expectations are met according to the risk identified (4). When feasible, using the method early in development can help identify any issues, generate data, and provide confidence the method is suitable for the intended use. When the alternative method is performed by a contract testing organization, ensure that the appropriate validation and comparability has been performed when appropriate and ethical. In addition, all the relevant data should be available to be reviewed by a regulatory body either through a drug/biologics master file (D/BMF) when possible or through other processes. Additionally, seeking early feedback from regulators, for example, through the CBER Advanced Technology Team, Innovation Task Force, or the INTERACT programs regarding the use of alternate technologies, is highly encouraged by the agencies.
Conclusion
References
- Senior, M. Fresh from the biotech pipeline: fewer approvals, but biologics gain share. Nat Biotechnol 41, 174-182 (2023). https://doi.org/10.1038/s41587-022-01630-6.
- Barone, P.W., Wiebe, M.E., Leung, J.C. et al. Viral contamination in biologic manufacture and implications for emerging therapies. Nat Biotechnol 38, 563–572 (2020). https://doi.org/10.1038/s41587-020-0507-2.
- ICH Topic Q5A (R1). Quality of Biotechnological Products: Viral Safety Evaluation of Biotechnology Products Derived from Cell Lines of Human or Animal Origin (CPMP/ICH/295/95), October 1997.
- ICH Topic Q5A (R2). Quality of Biotechnological Products: Viral Safety Evaluation of Biotechnology Products Derived from Cell Lines of Human or Animal Origin November 2023.
- World Health Organization (WHO) 2014. Guidelines on the quality, safety, and efficacy of biotherapeutic protein products by recombinant DNA technology. TRS 987, Annex 4.
- European Pharmacopoeia 5.2.3 Cell Substrates for the Production of Vaccines for Human Use.
- US FDA Guidance for Industry: Characterization and Qualification of Cell Substrates and Other Biological Materials Used in the Production of Viral Vaccines for Infectious Disease Indications (2010).
- ICH Topic Q7A. Good Manufacturing Practice Guide for Active Pharmaceutical Ingredients (CPMP/ICH/4106/00), November 2000.
- ICH Q2(R1) Validation of Analytical Procedures: Text and Methodology Guidance for Industry. US FDA September 2021.