New Tech Disrupts Traditional Aseptic Processing

There have been recent innovations around isolator and restricted access barrier system (RABS) technology. At the 2018 PDA Annual Meeting, session "B2: Aseptic Processing Isolators" will address these technologies on Tuesday, March 20 at 1:45 p.m. Below are two articles from each of the speakers.
Manufacturer Goes Gloveless on Filling Line
In aseptic processing, people are the primary source of contamination. Less human contact during manufacturing means less risk of contamination. To address this issue, one aseptic manufacturer successfully implemented a robotic filling line that operates in a closed isolator environment with no glove ports. This case study takes a look at the technology involved and highlights the implementation team’s experience with installation and qualification.
Look Ma, No Gloves!
The firm installed the Vanrx SA25, a gloveless robotic aseptic filling isolator (or aseptic filling “Workcell”), which consists of two isolators separated by a passthrough-style door. Sterile, ready-to-use (RTU) components are loaded in the biodecontamination and staging isolator (DSI); then, filling and closure operations take place downstream in the filling isolator. The SA25 WorkCell can fill nested syringes, vials and cartridges. All material handling, filling and closing activities are performed by robotics within the closed system.
Internal surfaces of the the DSI and filling isolator do not come in contact with product, and they are biodecontaminated using vapor-phase hydrogen peroxide (VPHP). A validation strategy was developed to ensure the biodecontamination process provides a spore logarithmic reduction ≥6 and reduces residual hydrogen peroxide to 1 ppm at the end of aeration.
The horizontal, unidirectional airflow within the filling isolator is environmentally monitored by viable and nonviable sampling. Nonviable sampling uses a standard particle-counter system, whereas viable sampling is customized. Viable samples are collected periodically throughout a filling batch via a rotary centrifugal air sampler (RCS). The RCS unit provides sterile docking and undocking to/from the isolator through an integrated Getinge 190mm Beta Flange and a robotic arm inside the filling isolator actuates the alpha/beta door during sampling. A separate “labscale” isolator is required to create a sterile, Grade A environment for RCS preparation (e.g., decontamination and agar strip insertion) and for subsequent agar strip removal.
The filling system consists of a single needle, gamma-irradiated, fully disposable fluid pathway and a peristaltic pump. The filling operation consists of the following sequence:
- Biodecontamination of full system (DSI and filling isolators)
- Manual debagging of RTU components (e.g., syringes, vials, closures, etc.)
- Manual loading of RTU components inside DSI
- Biodecontamination of DSI and external surfaces of RTU primary packaging
- Transfer of RTU component tub from DSI to filling isolator
- Tub lid and liner removal
- Detub
- Filling
- Vial closure by compression or syringe closure by vacuum
- Retub of filled units (e.g., nest placed back into tub)
- Transfer of finished tub back into DSI
- Unloading of DSI following completion of last fill (tub)
The process repeats until the desired quantity is filled.
Validation: Time-Consuming But Critical
Onsite installation involved uncrating, movement to the filling suite and tying in to utilities (instrument air, process air, electrical and exhaust). The SA25 Workcell is relatively small (13.5’x6’x8’), so moving the equipment into the filling suite was straightforward and required no major disassembly. In fact, the isolator was moved from the first to the fourth floor of the building using a standard freight elevator. The isolator was then installed in a Grade C/ISO 8 filling suite with sufficient space to accommodate staging of commodities and filled units. Even with this allowance, the Workcell only took up 560 feet2 of room space.
Validation followed the standard approach of factory acceptance testing, site acceptance testing, installation qualification, operation qualification and performance qualification. Key components included automation validation, filling and machinability validation, biodecontamination cycle validation, and environmental monitoring validation. The most time consuming activity? Development and validation of the biodecontamination cycles.
The SA25 Workcell consists of three biodecontamination cycles: full system, DSI and filler. The full system cycle is carried out at the beginning of a batch before loading the DSI, which serves two purposes: decontamination of the filling isolator and decontamination of the transfer door gasket that separates the DSI and filling isolators. Following the full system biodecontamination cycle, the DSI is loaded and the DSI biodecontamination cycle is carried out. The filler biodecontamination cycle is only used if the filler requires decontamination (e.g., sterility of the filling isolator is breached).
In general, the biodecontamination cycle comprises four process steps: leak test, preheating, decontamination and aeration. The process does not include a step to condition air inside the chamber. The temperature and humidity of the air within the isolator depends on room conditions at the start of the cycle and must be strictly monitored and controlled. No air flows into or out of the isolators during the biodecontamination process; internal fans are used to help distribute the VPHP during the exposure period. External HEPA-filtered air is introduced only during aeration.
The biodecontamination validation strategy included development activities (conducted via engineering studies) to establish critical process parameters and biological indicator placement locations. Baseline parameters were derived from vendor-executed microbiological studies. Key components of the development included:
- Dosing confirmation of the hydrogen peroxide
- Temperature mapping
- Chemical indicator mapping
- Biological indicator characterization and fractional studies
- Biological indicator mapping to locate worst-case locations
- Testing for residual H2O2 (e.g., inside commodity tubs and isolators)
The performance qualification occurred subsequent to confirmation runs conducted during development and included biological indicator studies as well as aeration evaluations.
An overview of the project timeline and completed suite is provided in Figures 1–4.
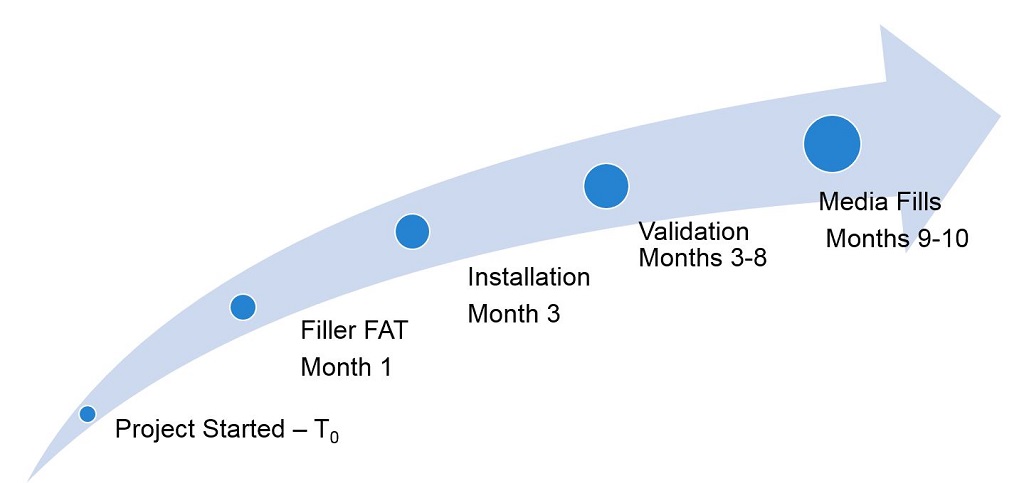
Figure 1 Project Timeline
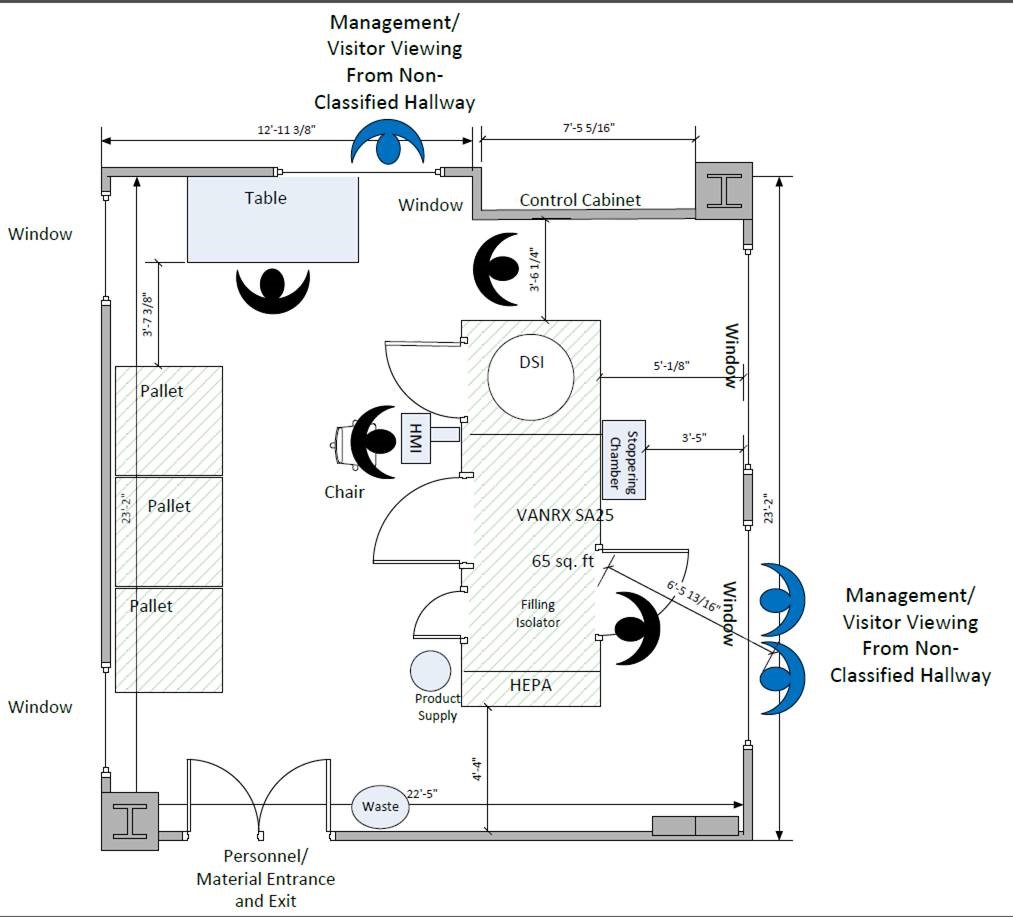
Figure 2 Room Layout

Figure 3 Completed Filling Suite
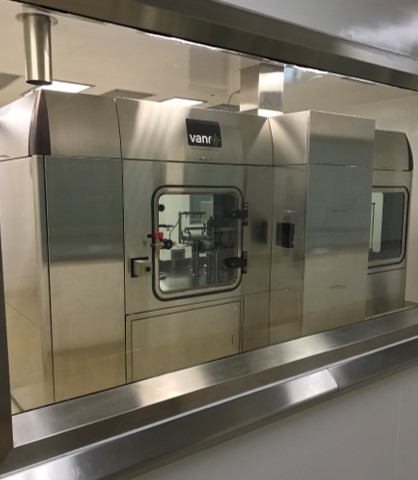
Figure 4 Filling Suite
New Tech, New Lessons Learned
This project introduced several new technologies to the site, which pushed the implementation team to think outside the box and collaborate with others in the industry. They quickly learned that having appropriate resources and internal support is essential for successful implementation.
As with any new project, more time was allotted for factory acceptance testing activities and to ensure that all cross-functional teams participated. At a minimum, a factory acceptance testing team should include representatives from the engineering, quality, maintenance and operations departments. The vendor played a key role in other studies. Vanrx executed several microbiological studies for the biodecontamination cycles and provided summary reports which were extremely helpful in establishing test functions and monitoring locations for development studies.
Communication was crucial to the successful validation of the Vanrx SA25 filler: good communications and strong working relationships between equipment vendors and end-users. The project team and management had to understand the inherent risk of setbacks involved when working with new technologies.
Robotic automation presented new challenges to the team who were unfamiliar with the technology. Robotic movements are precise and, if unaccounted for, dimensional variability of incoming commodities can greatly impact the fill operation (e.g., dimensional tolerances). Consequently, reviewing vendor specifications was essential. And, although the A25 Workcell is designed for full automation, some occasions required operator intervention—via robot movements. So operators must be well trained on the technology. The team found that those with a background in automation adapted most readily.
As robotics and automation improve, the human element will be removed entirely from isolator technology, greatly reducing the risk of contamination. Yet, humans will still play a vital role in the successful implementation and validation of new isolator technologies.
New Approach Enables Isolator/RABS Combination
Can restricted access barrier systems (RABS) and isolator cleanroom technology be combined to help achieve the highest level of quality and flexibility in aseptic processing? A new approach to decontamination opens up new possibilities for manufacturers, particularly contract development and manufacturing organizations (CDMOs), interested in using a process that pairs both technologies.
[Editor's Note: Watch the author go into more detail about the features of the system in a PDA Letter "On the Issue" video.]
To implement new technologies for the aseptic filling of injection systems, sterile drug manufacturers must first consider global regulatory requirements. This is especially true given the recent release of the EU Annex 1 revision. At the same time, the trend toward more specialized treatments necessitates greater flexibility when it comes to product format, packaging and filling needs. The competing needs for sterility and flexibility seem to force CDMOs to choose between isolators and RABS.
Isolators are generally able to meet the new regulatory requirements as isolator technology employs a fully sealed unit entirely separated from the outside environment. The technology relies on extensive decontamination processes, however, that require long downtimes for product changeovers. RABS technology, on the other hand, involves barrier and dynamic airflow separation between the environment and the drug product which offers the advantage of faster setup and efficient product changeover and flexibility.
The Best of Both Worlds
But what would happen if the high levels of sterility in isolators could be merged with the flexibility of RABS? Combining the two systems would result in faster cycle times, reducing downtime, expanding capacity and increasing flexibility. A new decontamination process recently developed does just that. This new process uses hydrogen peroxide (H2O2) to decontaminate an entire cleanroom prior to each batch, mitigating the risk of microbial contamination. The background environment—Laminar Air Flow area surrounding RABS—is Grade B, air class 100 (Figure 1).

Figure 1 Cleanroom Technology Uses an Automated Dispersion System Inside and Outside the Barrier
Because the system is closed and a fully automated dispersion system is integrated into the cleanroom technology, the H2O2 is not funneled into the cleanroom via the climate control system; most is evacuated by a separate ventilation system. Meanwhile, a system of stainless steel tubes built into the cleanroom walls aerosolizes the H2O2 solution into ISO 5 and 7 cleanrooms through dual-substance jets embedded in the ceiling and walls, inside and outside the barrier.
An entire cleanroom can be decontaminated in less than three hours—incredibly fast by current standards—and set up for aseptic processing immediately afterward (Figure 2). Overall, this system better prevents bacterial load than the isolator because the prep work is done behind closed barriers at all times in an aseptic environment following decontamination. This prep work requires that a steam-sterilized “liquid path” be brought into the barrier. Continuous online monitoring of several variables helps ensure the effectiveness of the decontamination process.
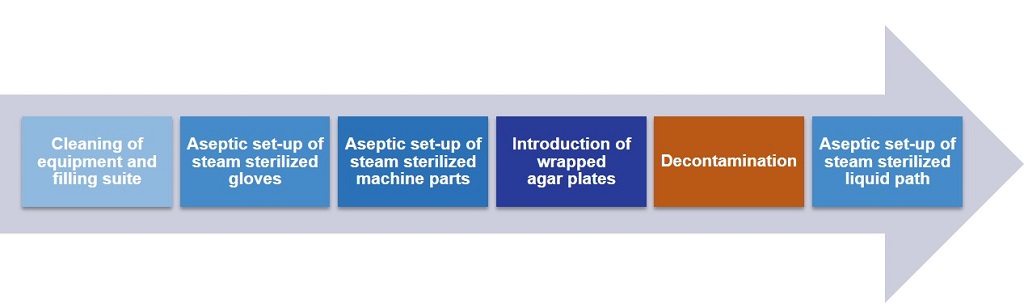
Figure 2 Decontamination Cycle for the Combined Setup
This unique approach to decontamination enhances the sterility of RABS while enabling the quick changeover of isolators. By combining the benefits of these two technologies, this new system achieves quality and safety standards that well exceed cGMP. It also promises a faster cycle time that reduces filling line downtime, and increases operational capacity and provides greater flexibility for customers. This blend of conventional processes and new technology supports the need for sterility and flexibility that represents the future of manufacturing.