PDA Survey Results: Current State of Cryopreservation
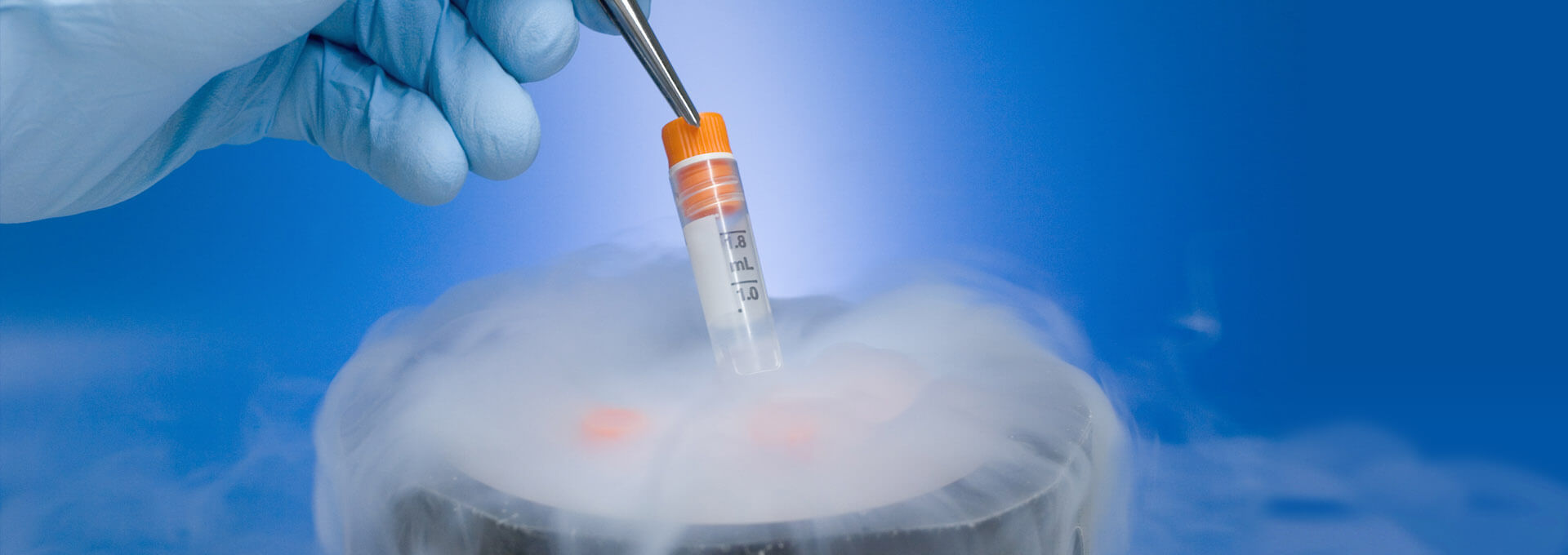
The first successful cryopreservation studies were performed in 1949 (1), and since then, numerous strategies have been proposed and adopted to facilitate the rapid restoration of cellular function following freezing. Unfortunately, despite decades of research and increasing emphasis in the clinic, harmonized cryopreservation standards for cell and gene therapies (Advanced Therapy Medicinal Products or ATMPs) do not exist, and cryopreservation protocols vary widely in practice both between, and even within, commercial and academic groups.
To address the state of cryopreservation protocols currently employed, PDA designed and distributed the survey Cryopreservation Practices and Experiences for members to share their experiences pertaining to biological handling practices, the results of which are summarized here.
The survey was divided into a general overview, sections on the generation and monitoring of cell banks, and questions related to troubleshooting and resolution.
Since 32% of the respondents’ products combine cryopreservation with nonfrozen storage and shipment at refrigerated temperatures, the term “biopreservation” was adopted for the survey unless discretely specified.
Overall, biopreservation was primarily incorporated into product commercialization activities (38%), followed closely by clinical (31%) and research (24%) activities.
Storage temperature varied widely among respondents, which reflected the diversity of biological handling requirements, with liquid nitrogen (LN2; -196 °C to -140 °C) being the most common (42%), followed by -80 °C (30%) and 2–8 °C (12%). In practice, only temperatures below the glass transition of water [Tg; ~-135 °C] (2) would completely arrest molecular motion and permit long-term storage of cells in banks (3). Storage temperatures above Tg would allow for gradual degradation and should only be employed for cellular products as a short-term storage solution.
Despite the importance of temperature maintenance, a plurality of respondents (50%) employ biopreservation only for the final cell product and not for the incoming biological source material (24%). Incoming cellular material that does not incorporate appropriate biopreservation controls can experience significant cell loss or a reduction in functionality, which may adversely affect downstream manufacturing and final product potency (4). Temperature excursions are most commonly recorded using data-loggers (52%) or monitored by a combination of data-loggers and real-time monitoring (30%). Most biopreserved material is transported in insulated thermal shippers containing dry (~-80 °C) or wet ice (72%).
In commercial and research practice, most cell banks were generated in a self-identified “low-density” (69%) cell concentration (<2 x 107 cells/mL density) in plastic cryovial format (67%). At least some form of “practice” banking was performed by a majority of groups during which cryopreservation protocols were optimized for a given cell type (57%). Container closure integrity protocols varied considerably among most groups but centered primarily on a dye-ingress assay. Similarly, the cryopreservation process varied in length from immediate freezing (LN2 immersion) to slow cooling over 8 hours. And, while process pause steps were not typically validated as part of the workflow (65%), a large majority (73%) incorporated stability studies to ensure the cryopreservation process did not impact sample integrity.
Despite the availability of commercial biopreservation media, a number of respondents employ a combination of commercial media with a form of “home brew” formulated in-house (42%) or commercial media alone (39%) that is specifically optimized for each cell line (78%). When commercial media was chosen, it was primarily manufactured under good manufacturing practice (GMP) guidelines (86%). Commercial cryopreservation media was largely devoid of animal/human proteins (54%) and employed dimethyl sulfoxide (DMSO) as the primary cryoprotectant (85%). Controlled rate LN2-based freezing devices were the most common method of sample freezing (43%), although generic protocols were primarily adopted (55%) without an optimized freezing rate that forces ice nucleation at a specified temperature (64%). For 76% of respondents, frozen samples are typically moved from the freezing device to vapor phase LN2 with the aid of dry ice to maintain the temperature of <-80 °C during transit. Cryopreserved samples are primarily thawed using manual methods (76%) in some form of water bath. Cryopreservation media is largely removed from the sample via centrifugation or wash prior to patient delivery (52%), although many respondents reported that the cryopreservation media is validated for infusion as an excipient, i.e., without wash (48%). Determination of post-thaw viability and functionality was application-specific, but most groups considered cryopreservation success to be viability greater than 75% across multiple cell bank thaws (91%).
This survey provides valuable insight into the current state of biopreservation with regard to biopharmaceutical and cell therapy manufacturing. In summary, biopreservation practice varied widely among survey respondents, which reflects both the relative infancy of cell usage in clinical product development and the lack of cryopreservation standards by regulatory bodies and professional organizations. Nonetheless, several commonalities can be surmised from the PDA’s cryopreservation survey.
In practice, biopreservation was largely adopted by commercial groups as part of the final product configuration for storage and transportation at frozen temperatures using LN2-based infrastructure. Procedurally, cryopreservation was performed using an LN2-based active freezer, and samples were thawed manually using a water bath.
Reflecting the need for appropriate quality assurance, a large majority of respondents sourced commercial cryopreservation media manufactured under GMP guidelines that was devoid of animal or human proteins and contained DMSO as the principle cryoprotectant. And finally, post-thaw viability was primarily determined by membrane integrity with a benchmark of >75% immediately post-thaw, but methods and functional measurements were specific to each group and application.
Despite these apparent commonalities, several potential deficiencies were revealed that may reduce the health and effectiveness of cells following storage. The primary cause of concern is that current practice primarily focuses only on the final product, as opposed to appropriate biopreservation controls being incorporated as a continuum throughout the product lifetime, including at the creation of the critical master and working cell banks. This is especially true of the incoming biological raw material, improper biopreservation of which could lead to excessive degradation and could impact all subsequent downstream steps in the product workflow.
Indeed, poor quality cellular starting material may propagate undesirable genetic traits that could negatively affect all aspects of downstream processing and compromise the final cellular product. Additional biopreservation protocols largely absent in current practice that may improve post-thaw cell health and function include, but are not limited to, cell-specific freezing/thawing rates, inclusion of a prebiopreservation incubation hold step, temperature of cryoprotectant addition and regulation of ice nucleation (4). Inadequate control over these variables may introduce undue sample variability and compromised post-thaw functionality. Indeed, more than half of the respondents (52%) experienced at least one incidence of poor post-thaw cellular function due to an unknown cause (69%), which emphasizes the importance of suitable biopreservation controls throughout the manufacturing process, from incoming raw material through final patient delivery.
PDA is taking a lead role in helping both commercial and clinical groups with their cryopreservation efforts. To better harmonize ATMP cryopreservation practices, the Association convened a working group comprising experts from academia, industry and governmental regulatory bodies to compile and draft current best practices into a single reference document.
The efforts of this PDA working group resulted in a document entitled, Cryopreservation of Cells for Use in Cell Therapies, Gene Therapies, and Regenerative Medicine Manufacturing: An Introduction and Best Practices Approach on How to Prepare, Cryopreserve, and Recover Cells, Cell Lines, and Cell-Based Tissue Products that was submitted in July 2020 for consideration as American National Standards Institute (ANSI) standard. In doing so, PDA continues its ongoing mission to advance pharmaceutical and biopharmaceutical manufacturing science and regulation so members can better serve patients and provide critical guidance to hasten the translation of promising ATMP products from the bench to the bedside.
References
- Polge C, Smith AU, Parkes AS. Revival of spermatozoa after vitrification and dehydration at low temperatures. Nature. 1949;164(4172):666. doi:10.1038/164666a0
- Chen T, Fowler A, Toner M. Literature review: Supplemented phase diagram of the trehalose-water binary mixture. Cryobiology. 2000;40(3):277-282. doi:10.1006/cryo.2000.2244
- Hubel A, Spindler R, Skubitz APN. Storage of Human Biospecimens: Selection of the Optimal Storage Temperature. Biopreserv Biobank. 2014;12(3):165-175. http://online.liebertpub.com/doi/abs/10.1089/bio.2013.0084.
- Hawkins BJ, Abazari A, Mathew AJ. Biopreservation Best Practices for regenerative medicine GMP manufacturing & focus on optimized biopreservation media. Cell Gene Ther Insights. 2017;3(5):345-358. doi:10.18609/cgti.2017.035